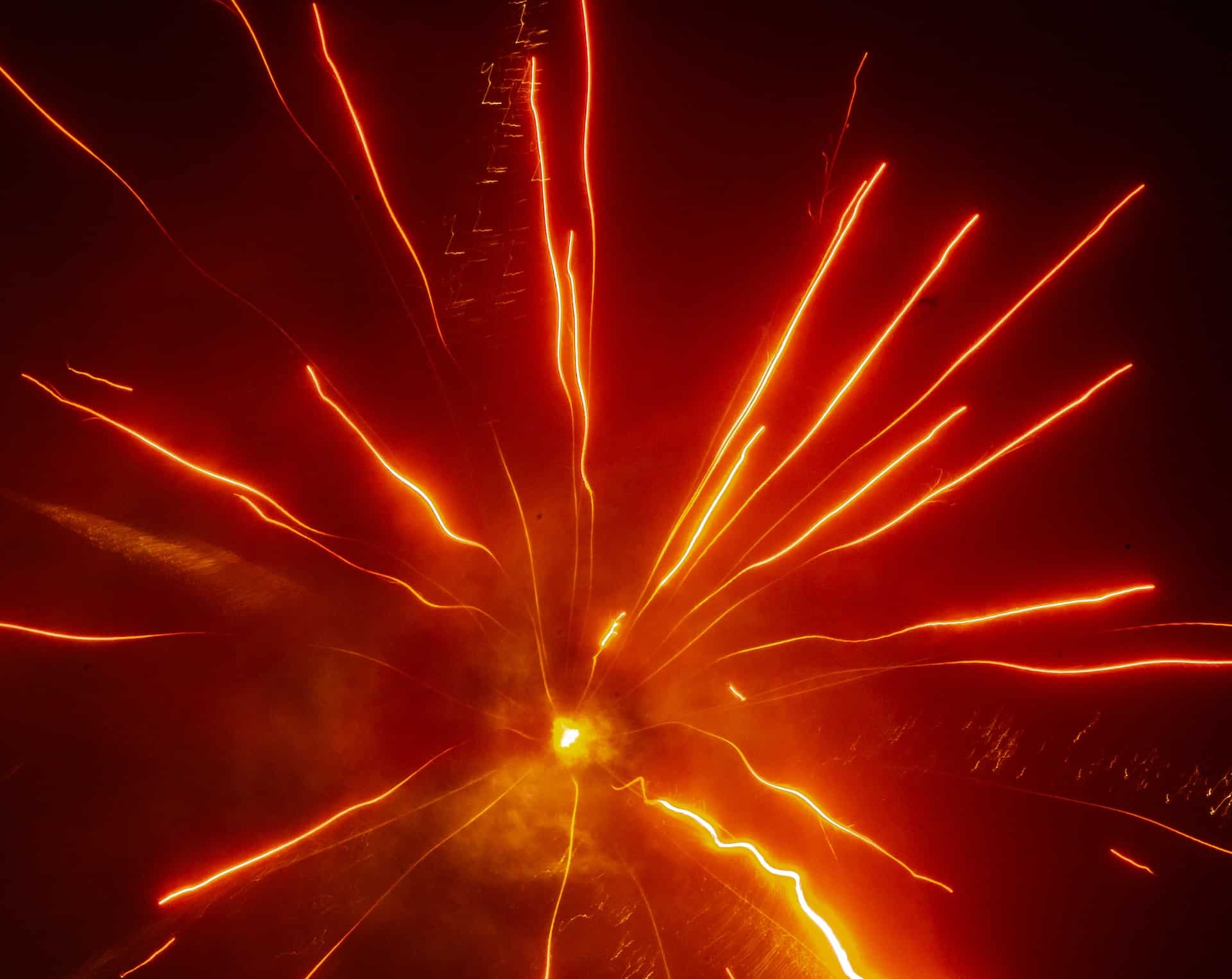
J. Robert Oppenheimer’s Legacy in Quantum Mechanics
October 5, 2023 | Quantum Computing Updates
Article by: Law Clerk Radhi Shah
The release of the film “Oppenheimer” in July 2023 has brought renewed attention to the renowned scientist J. Robert Oppenheimer’s life. While Oppenheimer is primarily remembered as the key figure behind the development of the atomic bomb, his early contributions to the field of quantum mechanics have had a lasting impact on modern quantum chemistry. His groundbreaking work on molecular quantum mechanics remains pivotal in my teaching and research as a physical chemist.
One of Oppenheimer’s significant achievements in the realm of quantum mechanics was the formulation of the Born-Oppenheimer approximation, detailed in a 1927 paper co-authored with his research mentor Max Born.
Although the theory is often attributed to both Oppenheimer and Born, most historians recognize that the majority of the work was carried out by Oppenheimer.
The Born-Oppenheimer approximation offers a method to simplify the intricate task of describing molecules at the atomic level. Imagine attempting to calculate the optimal molecular structure, chemical bonding patterns, and physical properties of a molecule using quantum mechanics. This involves defining the positions and movements of all atomic nuclei and electrons and computing the crucial charge interactions between these particles within the molecule.
Quantum-level molecular property calculations become even more intricate as particles exhibit wave-like behaviors and their precise positions cannot be determined. Instead, particles, particularly electrons, are described by wave functions that indicate their probabilities of being in specific regions of space. Solving the molecular Schrödinger equation, which involves determining these wave functions and the corresponding molecule energies, presents a formidable challenge. In fact, this equation cannot be solved exactly even for the simplest molecule, H₂⁺, consisting of just three particles: two hydrogen nuclei (protons) and one electron.
Oppenheimer’s approach introduced a way to obtain an approximate solution to this problem. He observed that atomic nuclei are much heavier than electrons, with a single proton weighing nearly 2,000 times more than an electron. Consequently, nuclei move significantly slower than electrons, enabling scientists to treat them as nearly stationary objects while solving the Schrödinger equation exclusively for electrons. This simplifies the calculation’s complexity and facilitates the determination of the molecule’s wave function.
The significance of the Born-Oppenheimer approximation extends beyond streamlining quantum mechanics calculations for molecules; it fundamentally shapes chemists’ understanding of molecules and chemical reactions.
Scientists often visualize molecules as fixed nuclei with shared electrons that move between nuclei, leading to the familiar “ball-and-stick” models used in chemistry education. This perspective is a direct consequence of the Born-Oppenheimer approximation.
Moreover, this approximation influences how scientists conceptualize chemical reactions. During a chemical reaction, atomic nuclei undergo rearrangements and motion, guided by electron interactions that establish an energy surface for the nuclei to traverse throughout the reaction. In essence, electrons drive the progression of a chemical reaction. Oppenheimer’s work elucidated that electron behavior lies at the heart of chemistry as a scientific discipline.
Over the past century, scientists have made significant advancements in computational quantum chemistry, aided by the availability of faster and more powerful high-end computing resources. This field has seen extensive growth and applications, ranging from the discovery of novel pharmaceuticals to the design of improved photovoltaic materials, all heavily reliant on the Born-Oppenheimer approximation.
However, it’s important to note that the Born-Oppenheimer approximation is not without limitations. It may break down in specific scenarios, such as light-driven chemical reactions, as seen in the process enabling vision in animals. Chemists are actively exploring solutions for these cases. Nevertheless, the application of quantum chemistry made feasible by the Born-Oppenheimer approximation will continue to expand and improve. Looking ahead, the emergence of quantum computers holds the promise of enhancing computational quantum chemistry by enabling faster calculations on increasingly complex molecular systems, ushering in a new era for this field.